The Three-Dimensional World of Molecules: Molecular Geometry and Molecular Shapes
- Emre Can Buluz
- Apr 28
- 7 min read
The three-dimensional structure of molecules is one of the most important factors determining their chemical and biological properties. Molecular geometry is the field that studies the arrangement of atoms in space and their bond angles. This geometry varies depending on factors such as the hybridization states of the atoms, the types of bonds, and the electron pairs around the central atom. Models such as VSEPR (Valence Shell Electron Pair Repulsion) theory are widely used to predict molecular shapes.
VSEPR Model
There are two general rules for using the VSEPR model:
In terms of electron pair repulsion, double bonds and triple bonds can be treated like single bonds. This approach is a good approximation for qualitative purposes. However, it is important to remember that in reality, multiple bonds are "wider" than single bonds; that is, when two or three bonds are between two atoms, the electron density takes up more space.
If a molecule has two or more resonance structures, we can apply the VSEPR model to any of them. Formal charges are usually not shown (1).
According to the VSEPR model, if there are only two electron pairs in the valence shell of an atom, these pairs are positioned as far away from the nucleus as possible to repel each other to a minimum. This causes the electron pairs to take a linear arrangement. In other words, the electron pairs occupy spaces that are 180° apart (Figure 1.).

If an atom has three electron pairs in its valence shell, these pairs tend to be arranged in a plane, oriented toward the corners of an equilateral triangle. This arrangement is called trigonal planar, where the regions occupied by the electron pairs are oriented at 120° angles to each other (2) (Figure 2.).
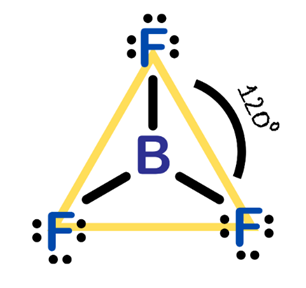
When an atom has four electron pairs in its valence shell, these pairs are arranged tetrahedral. That is, if you imagine the atom at the center of a regular tetrahedron, each electron pair is usually oriented toward a corner (or vertex). In this case, the regions occupied by the electron pairs are oriented at approximately 109.5° angles to each other (Figure 3.).

Large atoms such as phosphorus can have more than eight valence electrons. The phosphorus atom in the phosphorus pentachloride (PCl₅) molecule has five electron pairs in its valence shell. When there are five bonding pairs around the phosphorus, the PCl₅ molecule has a trigonal bipyramidal geometry. However, not all corners of the trigonal bipyramidal structure are equal, that is, the angles between the electron pairs are not exactly the same. Therefore, the directions in which the electron pairs are oriented are not equal. Two of these directions are called axial directions and form an axis through the central atom. These axial directions are separated by 180° from each other. The other three directions are called equatorial directions. These directions are oriented to the corners of an equilateral triangle lying on a plane passing through the central atom and perpendicular (90°) to the axial directions. The equatorial directions are separated by 120° from each other (Figure 4.) (2).
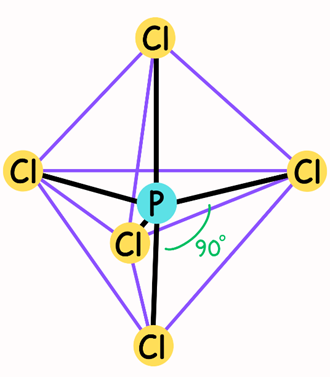
The most stable arrangement of six S–F bonds is an octahedral shape (Figure 5). An octahedron has eight faces. This structure is formed by joining two square pyramids with a common base. In this case, the central atom (i.e., sulfur, S) is at the center of the square and the surrounding atoms are at the six corners. All bond angles are 90°, but the bond angle between the central atom and pairs of atoms in diagonally opposite directions is 180°. Since all six bonds are equivalent in an octahedral molecule, the terms axial and equatorial cannot be used as in trigonal bipyramidal molecules (Figure 5) (1).
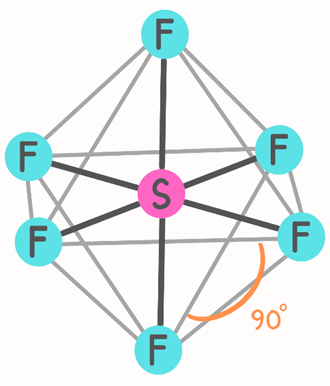
The Relationship Between Hybridization and Molecular Geometry
The relationship between hybridization and molecular geometry is a fundamental concept in understanding the bonding structure and spatial arrangement of atoms. Hybridization is the process by which orbitals in the energy levels of atoms mix to form new hybrid orbitals, and the orientation of these orbitals determines the geometry of the molecule.
sp³ Hybridization
Hybrid orbitals are new orbitals formed by mixing different orbitals. The idea of combining these orbitals was proposed by Linus Pauling in 1931 (3). If one s and three p orbitals in the second shell of carbon are combined into four equal orbitals, each orbital will have one part s and three parts p character. Such a hybrid orbital is called a sp³ hybrid orbital. Each hybrid orbital has 25% s character and 75% p character. These four orbitals are degenerate, meaning they have the same energy level (4).

Like a p orbital, an sp³ hybrid orbital has two lobes. However, these lobes differ in size because the s orbital contributes to one lobe of the p orbital and subtracts from the other. The larger lobe of the hybrid orbital is used in bond formation.
sp2 Hybridization
In order to bond with three atoms, each carbon hybridizes three of its atomic orbitals. Since three orbitals (one s orbital and two p orbitals) are hybridized, three hybrid orbitals are obtained. These orbitals are called sp² hybrid orbitals. An sp² hybridized carbon atom is called a trigonal glider carbon because it is bonded to three atoms defining a plane. The unhybridized p orbital is perpendicular to the plane defined by the axes of the sp² orbitals. The carbons of ethene form two bonds with each other. This is called a double bond. The two carbon-carbon bonds in a double bond are not the same. One of the bonds is formed by the overlap between the orbital of one carbon and the orbital of the other carbon; This is a sigma bond because it is formed by end-to-end overlap. Each carbon forms the bonds by overlapping the s orbital of hydrogen using its other two orbitals. The second carbon-carbon bond is formed by the side-by-side overlap of two unhybridized p orbitals. P orbital bonds formed by side-by-side overlap are called pi bonds (4).

The two p orbitals must be parallel to each other for maximum overlap to form a pi bond. This requires that the triangle formed by one carbon and two hydrogens be in the same plane as the triangle formed by the other carbon and two hydrogens. This means that all six atoms of ethene are in the same plane, and the electrons in the p orbitals fill a space above and below this plane (4).
sp Hybridization
Each of the carbon atoms in the molecule of ethene (also called acetylene) is bonded to only two atoms, one hydrogen and one other carbon. Since each carbon forms a covalent bond with two atoms, only two orbitals (one s and one p) are hybridized. This results in two equivalent sp orbitals. Therefore, each carbon atom in ethene has two sp orbitals and two unhybridized p orbitals. One of the sp orbitals of one carbon in ethene overlaps the sp orbital of the other carbon, forming a carbon-carbon sigma bond. The other sp orbital of each carbon overlaps with the s orbital of a hydrogen, forming the carbon-hydrogen sigma bond.
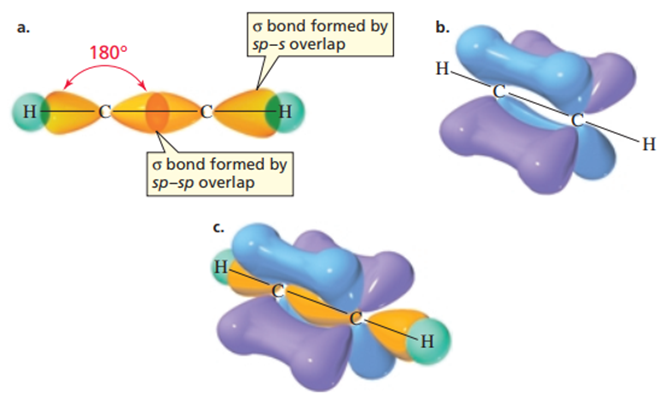
To minimize electron repulsion, the two sp orbitals are oriented in opposite directions. As a result, the bond angles are 180°. The two unhybridized p orbitals are perpendicular to each other, and both are perpendicular to the sp orbitals. Each unhybridized p orbital overlaps side by side with a parallel p orbital on the other carbon, forming a pi bond. The result is a triple bond consisting of one sigma bond and two pi bonds. Since the two unhybridized p orbitals on each carbon are perpendicular to each other, regions of high electron density are formed above, below, in front of, and behind the internuclear axis of the molecule. The potential map for the ethyne molecule shows that the negative charge accumulates in a cylindrical region surrounding the egg-shaped molecule (4).
As a result, the relationship between hybridization, molecular geometry, and molecular shapes plays a critical role in understanding the three-dimensional structure and behavior of chemical compounds. The type of hybridization of an atom determines the orientation of the bonds in space, shaping the geometry of the molecule, which directly affects the physical and chemical properties of the molecule. When considered within the framework of VSEPR theory, the repulsive forces between electron pairs are also an important factor determining the final shape of the molecule. Therefore, hybridization, molecular geometry and molecular shapes should be considered together to understand the properties of molecules such as their reactivity, polarity and interactions.
REFERENCES
1. Raymond Chang, Jason Overby. (2011). General Chemistry: The Essential Concepts. McGraw-Hill.
2. Darrell Ebbing, Steven D. Gammon. (2007). General Chemistry: Media Enhanced Edition. Cengage Learning.
3. Linus Pauling. (1931). The nature of the chemical bond. Application of results obtained from the quantum mechanics and from a theory of paramagnetic susceptibility to the structure of molecules. J. Am. Chem. Soc. 53.
4.Bruice, Paula Yurkanis. (2004). Organic Chemistry. Upper Saddle River, NJ :Pearson/Prentice Hall.
Comments